Detection and surveillance of gastric cancer precursors: evolving guidelines and technologies
Introduction
Background
Gastric cancer causes significant morbidity and mortality worldwide. In 2020, there were an estimated 1 million new cases of gastric cancer and 770,000 gastric cancer deaths worldwide (1). In the United States (US), there were 26,380 estimated new cases of gastric cancer, and approximately 11,090 deaths in 2022 (2). In the US, 5-year survival is 32% across stages (2); moreover, fewer than one-third of cancers are diagnosed at a localized stage (3). As such, the majority of gastric cancers in the US are diagnosed at later stages, often with metastases, and with poor prognosis (4). Early detection of gastric cancer and pre-neoplastic lesions continues to be a challenge for clinicians.
The majority of cancers in the stomach are adenocarcinomas (90–95%). Other types of cancers which occur in the stomach include gastric lymphomas, gastrointestinal stromal tumors, and neuroendocrine tumors (5). Gastric adenocarcinomas are further divided based on histology, as intestinal and diffuse types, and based on anatomic location, as cardia and non-cardia gastric cancers (6). This review will focus on non-cardiac gastric adenocarcinoma (NCGA), the cancer for which the etiologic role of precursor lesions is most clearly established.
Rationale and knowledge gap
Currently, there are multiple endoscopic techniques and technologies to detect and monitor gastric precursor lesions, as well as evolving surveillance guidelines from various international societies. Given the advancement in endoscopic imaging modalities and a recent trend towards early detection and management of precursors, we aim to analyze current literature and highlight overarching themes. Through this review, we discuss imaging modalities for precursor detection and risk stratification, and propose a novel management algorithm for dysplasia based on current literature.
Objective
This review was conducted to provide an overview on current endoscopic modalities to detect NCGA precursors and evaluate recently published surveillance guidelines from various societies.
Precursor lesions
The transition from normal mucosa to NCGA is believed to occur through a stepwise cascade of precursors of increasing severity, known as Correa’s cascade (7). In this precancerous pathway, the normal gastric mucosa is subject to inflammation, as seen by the infiltration of polymorphonuclear neutrophils and mononuclear leukocytes on biopsy (7). Both worldwide and in the US, the leading cause of this inflammatory insult is chronic infection by Helicobacter pylori (Hp); it has been estimated that 89% of NCGAs were associated with Hp infection globally (8). However, in the US and other Western nations there is also a moderately high prevalence of autoimmune gastritis. Autoimmune gastritis represents an alternative pathway to carcinogenesis independent of Hp, and is characterized by reduced gastric acid secretion, elevated gastrin levels, enterochromaffin-cell hyperplasia, and in certain cases development of anti-parietal and anti-intrinsic factor antibodies (9-11). Pernicious anemia may be a late manifestation of autoimmune gastritis and may be a marker of increased cancer risk.
In both Hp-induced and autoimmune gastritis, the prolonged inflammatory state results in the loss of normal glandular structure and thinning of the mucosal layer, termed atrophic gastritis (AG) (12). The atrophy of glands can eventually be replaced by fibrosis, connective tissue, or other intestinal-type epithelial cells (7,9). The next stage is the development of gastric intestinal metaplasia (GIM), which can be pathologically recognized by the presence of goblet cells, absorptive cells, and Paneth cells (12). Histologically, GIM can be classified as either complete or incomplete. Complete GIM is characterized by small intestine-type epithelial cells with a well-defined brush border, goblet cells, and expression of small intestinal digestive enzymes. By contrast, incomplete GIM is characterized by colonic epithelium, the absence of a brush border, and loss of expression of certain digestive enzymes (7,13). It is believed that incomplete GIM has increased NCGA risk compared to complete GIM. In certain cases, GIM can advance to dysplasia, the penultimate step prior to NCGA development. Dysplasia can be broadly defined as the presence of neoplastic epithelium characterized by enlarged nuclei and cellular pleomorphism, but in the absence of invasion across the lamina propria (14). Once neoplastic cells violate the basement membrane and into the stroma, it is considered invasive carcinoma (7).
Endoscopic techniques of detection
Traditionally, white light endoscopy (WLE) has been utilized to visualize the gastric mucosa. However, there is a high degree of interobserver variability with WLE, which limits the reliability of differentiating neoplastic or pre-neoplastic gastric lesions based on conventional macroscopic visualization (15). Although there has been improvement in detection with the advent of high-definition WLE, GIM has few distinguishable macroscopic traits, which makes it particularly challenging to identify (16,17). Therefore, recent advances in technology have targeted alternative techniques to improve mucosal visualization and diagnosis of gastric lesions (18,19). In this section, we discuss the methodology and application of several advanced endoscopic imaging modalities to improve precursor detection.
Narrow-band imaging (NBI)
NBI is a method of image-enhanced endoscopy (IEE) that uses a filter to select a narrow spectrum of wavelength for blue (415 nm) and green (540 nm). Hemoglobin absorbs blue and green light specifically, thus allowing for enhanced visualization of the superficial mucosa, submucosal vessels and capillaries through this technique (20). Pimentel-Nunes et al. proposed a simplified classification of gastric lesions with NBI (21,22). In this classification, normal gastric mucosa is identified by a regular circular pattern surrounded by thick and regular vessels, in the antrum and body respectively. GIM is characterized by regular ridge pattern or tubulo-villous mucosa. Light blue crests are slightly raised areas with white-blue coloration that also suggest GIM (Figure 1) (23). Dysplasia can be characterized by irregular mucosa and vessels, and a white opaque substance (21,22).
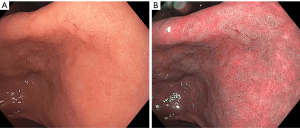
Multiple studies have shown the superiority of NBI over traditional WLE to accurately diagnose precursor lesions. One prospective blinded clinical trial with 112 study participants demonstrated that NBI examination improved diagnostic yield compared to high-definition WLE (65% vs. 29%) (24). Even in the setting of autoimmune gastritis (with its corpus-predominant inflammation), NBI examination appears to be highly sensitive for GIM detection (25). Another multicenter prospective study across five countries showed higher sensitivity for diagnosis of dysplasia with NBI compared to WLE (92% vs. 74%) (22). In addition to lesion identification, NBI has been shown to be able to predict advanced operative link on GIM (OLGIM) stages with a sensitivity of 89% and specificity of 95% (26). Advanced stages OLGIM are associated with increased risk of the development of gastric neoplasia (27).
Blue-laser imaging (BLI) and linked color imaging (LCI)
Recently, there have been advances in other IEE techniques. One modality is BLI (Figure 2). This system features two laser wavelengths in the blue spectrum (450 and 410 nm), producing brighter high-resolution images of the superficial mucosal microvessel structures (28-30). A brighter version of BLI has also been developed, termed BLI-bright (31). A recent randomized control trial showed the superiority in the detection of early cancer of BLI-bright mode compared to WLE (93% vs. 50%) (32).
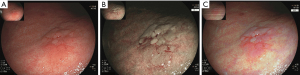
Another IEE modality is LCI. LCI technology utilizes blue, green and red color information and enhances the color contrast after processing (33). A major advantage of LCI is its brightness, which allows for excellent visualization of even distant mucosa—such an attribute is useful in a large lumen organ such as the human stomach (34). The stark color contrast makes early recognition of NCGA precursors easier, with early cancer having an orange-red color, and GIM having a purple color (33,34). One retrospective study comparing indigo carmine chromoendoscopy, BLI, and LCI showed that LCI was significantly better at identifying early differentiated cancers (35). Ono et al. demonstrated that the sensitivity of the “lavender color sign” for GIM with LCI was higher than the “whitish flat elevation” seen in WLE (91% vs. 19%) (36). Future studies will need to be performed to determine whether BLI and LCI can stratify the severity of precursor lesions.
Chromoendoscopy
Chromoendoscopy is a technique that applies topical chemical stains or pigments onto the gastrointestinal tissue. By adding the stain, there is enhanced visualization and localization of the mucosa (37). Chromoendoscopy has long been utilized by gastroenterologists to improve the visualization of the gastric mucosal surface. As early as 1969, Kimura and Takemoto used Congo red staining to define the atrophic border (separating AG from normal mucosa), and used the extent of this line (named the Kimura-Takemoto line) to define a scoring system for AG (38). In modern practice, spray catheters are utilized to deliver the pigment with an even distribution to the entire mucosal surface or a targeted region during endoscopy (39). A variety of staining agents can be utilized with chromoendoscopy and are classified based on their mechanism of action. The three categories are absorptive, contrast, and reactive stains (40).
The absorptive agents, such as methylene blue, are only actively absorbed across the cell membrane for specific cell types (40,41). For example, methylene blue selectively stains the epithelium in the small intestine and colon, as well as intestinal-type metaplasia located in esophageal and gastric mucosa (40-42). This allows for increased detection and evaluation of the anatomical extent of precursor lesions (41,43).
Contrast stains enhance the surface irregularities as the dye collects into the mucosal crevices. Indigo carmine is a commonly-used contrast stain that has a deep blue color (39,40). Studies have shown that indigo carmine combined with acetic acid had better diagnostic outcomes and identification of tumor borders compared to traditional endoscopy or chromoendoscopy with only acetic acid or indigo carmine (44,45). Acetic acid is a weak acid that splits disulfide bonds, thereby disrupting the mucous layer and causing a reversible intracellular protein denaturation. A white discoloration, known as the “acetowhite reaction”, occurs due to increased opacity of the surface and allows for improved visualization. This reaction is more prominent in the columnar epithelium, and dysplastic tissue reverses back to the red coloration more quickly than normal epithelium. Acetic acid chromoendoscopy has been shown to delineate the extent of GIM and dysplasia in the esophagus and cervix (46-48).
Reactive stains act by changing color after undergoing a chemical reaction with specific cellular components. Specifically, when Congo red dye is administered to the acidic gastric cells, it transforms from red to a dark blue or black color (39,40). Congo red can be used to detect achlorhydria, and it can also be utilized in conjunction with methylene blue to increase the rates of detection for early cancers (49-51). Dinis-Ribeiro et al. noted that magnifying chromoendoscopy with methylene blue had a sensitivity of 76% and 97% for diagnosis of GIM and dysplasia, respectively (43). A comparison study of 33 GIM patients showed a sensitivity of 64% for chromoendoscopy and 42% for standard endoscopy (41). In addition to screening for GIM, methylene blue can also be useful in predicting advanced OLGIM stages, with a sensitivity of 89% and specificity of 92% (52). Despite studies on the efficacy of chromoendoscopy showing promising results in terms of early detection and sensitivity, there have been practical limitations of its implementation, including the time-intensive nature of stain application during procedures (43-45,53).
Confocal laser endomicroscopy
Probe-based confocal laser endomicroscopy (pCLE) provides very high-resolution magnification of the gastrointestinal mucosa and tissue architecture at a cellular and subcellular level. A low power laser shines on the tissue at a specific depth and the reflection of fluorescent light passes through a pinhole to the detector. The pinhole allows the light from only one focal plane to be detected (54,55). Fluorescein dye is added either topically or intravenously. Topical fluorescein, such as acriflavine, stains the cell nuclei of the superficial epithelial layer. However, concerns of possible mutagenic effects have limited its use in clinical practice. Currently, intravenous fluorescein is most commonly used, due to its safety profile and ability to stain both the surface epithelium and the subepithelial layers (54-58). GIM can be identified with dark appearing goblet cells, villous-like foveolar epithelium, and columnar absorptive cells (Figure 3) (59).
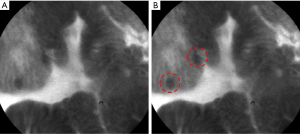
During an endoscopic procedure, a pCLE miniprobe is introduced through the accessory channel of a standard endoscope. This allows for increased flexibility, and simultaneous utilization of IEE techniques other than WLE (55,60,61). Thus, pCLE is advantageous as an “optical biopsy” that precludes the need for tissue excision. However, some limitations include the need for fluorescein administration, interobserver variability, and the initial learning curve for identifying mucosal features (62). Pittayanon et al. reported 80% accuracy after two teaching sessions for beginner learners. The beginner endoscopists underwent four different teaching sessions each 2-week apart, and accuracy was based on the number of correct answers from 20 different pre-selected pCLE images (63). When compared against other imaging modalities, one prospective comparison showed pCLE to be more sensitive (91%) in diagnosing GIM compared to conventional WLE (37%) and NBI (68%) (64). One meta-analysis of 23 Asian studies reported pCLE to demonstrate a pooled sensitivity of 91% for cancer, 92% for GIM, and 81% for dysplasia (65). In addition, pCLE is more precise in detecting the margins of early cancer during endoscopic submucosal dissection (ESD) compared to WLE with chromoendoscopy. Thus, pCLE is a good tool for screening gastric precursors and determining the extent of disease.
Auto-fluorescence imaging (AFI)
AFI detects the natural fluorescence from tissue. After exposure to different wavelengths of light, the endogenous fluorophores from the tissue (such as collagen, elastin, and porphyrin) emit longer wavelengths that can be detected. Each fluorophore has a distinct excitation and emission spectrum. In AFI, the tissue is exposed to excitation blue light and green light. The images from AFI are integrated and processed, such that the normal mucosa is seen as green and dysplastic mucosa as purple (66,67). The dysplastic tissue has reduction in auto-fluorescence due to the loss of collagen, increase in nucleus to cytoplasm ratio, and neovascularization (67,68). Some disadvantages of AFI include the large number of false positive results, and inability to visualize deeper levels of the tissue (69). Some experts suggest that AFI can be used to initially screen suspicious lesions and be used in combination with other imaging modalities.
Artificial intelligence (AI)
There has been much recent interest in the application of AI to improve both the diagnosis of precursor lesions and NCGA. AI utilizes computer algorithms with the ability to perceive, synthesize, and infer information to aid real-time endoscopic visualization after being trained on large annotated data sets (Figure 4). Recent trends and development show potential for high rates of image recognition accuracy in the field of gastrointestinal endoscopy. Integrating AI for image recognition could potentially mitigate the interobserver variability and learning curves of different endoscopic imaging modalities. For precursor lesions, one study demonstrated AI to have 92% sensitivity and 86% specificity for detecting GIM after being exposed to a training set of 1,880 NBI and magnified NBI images (70). Zhang et al. designed a neural network-based model trained on 3,042 AG images and 2,428 non-AG images, with an observed sensitivity of 95% and specificity of 94% for diagnosing AG (71). With regards to diagnosing cancers, Hirasawa et al. trained a convolutional neural network based on 13,584 images of cancer and evaluated the algorithm on 2,296 stomach images collected from 69 consecutive patients with 77 cancers (some individuals had synchronous tumors). In the validation set, the algorithm demonstrated 92% sensitivity (detecting 71 of 77 cancers), while also identifying 161 non-cancerous lesions as cancer (for a positive predictive value of 31%) (72). In addition, AI has been used to predict tumor invasion depth with great accuracy (above 90%) for multiple imaging modalities (73).
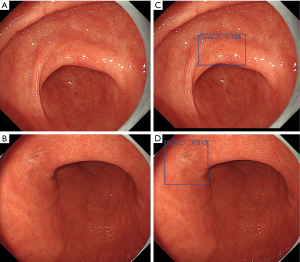
Surveillance
Until relatively recently, there were few guidelines to direct clinicians in the surveillance of gastric precursors. As a result, the decision to survey, and surveillance intervals, were highly variable across practices. However, over the recent few years a number of important clinical guidelines from both the US and Western Europe have begun to place gastric precursor surveillance within a normative framework. While containing modest differences, these guidelines serve to complement one another. Moreover, these guidelines uniformly recommended a stratified approach to surveillance focused on high-risk individuals, based on individual factors, anatomic extent, or histologic features.
Surveillance guidelines for GIM
In the US, the first guideline dedicated toward GIM management was published by the American Gastroenterology Association (AGA) in 2020 (74). While the guideline recommends against routine GIM surveillance endoscopy, high-risk individuals (specifically patients with incomplete or extensive GIM, family history of NCGA, racial/ethnic minority status, or immigration from high-incidence regions) may reasonably elect for endoscopic surveillance with 3–5 years intervals. It is important to note that the AGA guideline emphasizes the role of shared decision-making between physician and patient, adequate patient counseling on the potential but uncertain benefit of surveillance (cancer mortality reduction), as well as the potential risks of endoscopy.
In Europe, the “Management of epithelial precancerous conditions and lesions in the stomach” (MAPS II) guideline (16) was published in 2019, and represents an update to the original 2012 MAPS (75) statement. Individuals with single-site GIM with high-risk features (family history, incomplete GIM, persistent Hp) or patients with severe AG or GIM of both antrum and body should be offered surveillance endoscopy in 3 years. In addition, the British Society of Gastroenterology (76) and Italian multi-society (77) guidelines, both published in 2019, are concordant with the MAPS II guideline (76,77).
Compared to the AGA guideline which emphasizes shared decision-making, European guidelines offer more defined surveillance intervals based on individual-level risk factors (e.g., family history), disease extent, and histologic severity. By contrast, the US guideline emphasizes the role of race, ethnicity, and immigration history—perhaps reflective of the disease burden within the multi-ethnic US population. In whole, US and European guidelines serve to complement each other, and offer for the first time a normative framework for surveillance of gastric precursors.
Surveillance of dysplasia
A consensus is similarly emerging regarding the management of gastric dysplasia. The 2015 American Society for Gastrointestinal Endoscopy Standards of Practice document recommends close-interval (less than 1 year) surveillance of low-grade dysplasia (LGD) with topographic mapping, though a precise interval was not given (78). For high-grade dysplasia (HGD), prompt endoscopic or surgical resection is recommended (78). The European MAPS II guideline states that individuals with dysplasia in the absence of an endoscopically defined lesion should undergo immediate high-quality endoscopic reassessment by IEE. If a lesion is detected on this enhanced examination, prompt endoscopic resection and staging should be performed, as a significant number of dysplastic lesions may be upstaged to cancer on resection. If a lesion is not detected on second examination, Sydney protocol biopsies should be obtained (for AG/GIM staging), and endoscopic surveillance should be performed in either 6 months (for HGD) or 12 months (for LGD) (16). The British guideline recommends that patients with both non-visible LGD and HGD should undergo a second endoscopy, with IEE technique and extensive biopsy sampling. In cases of LGD, if no visible lesion is seen on this repeat examination, surveillance endoscopy should be performed within 1 year. If there is persistent, non-visible LGD seen, endoscopy should be performed annually thereafter. In cases of HGD, if no visible lesion is seen on this repeat examination and HGD persists, surveillance should be performed at 6-month intervals. Moreover, HGD should be discussed with a multi-disciplinary cancer team and referred to a clinician with appropriate expertise. Visible dysplasia should undergo en-bloc endoscopic resection; an endoscopic mucosal resection (EMR) technique can achieve en-bloc excision for lesions ≤10 mm in size, but only an ESD technique can ensure en-bloc excision for lesions >10 mm in size. The British guideline also suggests that a follow-up endoscopy 6 months after ESD or EMR of neoplasia, and if no subsequent lesions are identified, annually thereafter (76). Based on a synthesis of these guidelines, we propose a management algorithm for gastric dysplasia in Figure 5.
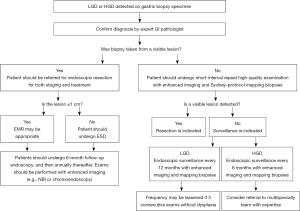
Future risk stratification strategies
Future molecular-based methods may revolutionize the practice of endoscopic surveillance of gastric precursors. One study based on a combined Dutch and Norwegian cohort assessed the role of family history, lifestyle factors, and several serologic biomarkers (including Hp antibody, gastrin-17, pepsinogen, and several pre-selected single nucleotide polymorphisms) on risk for progression (defined as upstaging of operative link score) over a median of 48 months of follow-up (79). The minor allele (C) on TLR4 (rs11536889) was inversely associated with progression of GIM. In a large Singaporean cohort study of a high-risk ethnic Chinese population, several gastric tissue genomic features, including shortened telomere length and chromosomal alterations, were associated with increased risk of progression from GIM to subsequent dysplasia or NCGA (80). Both germline and somatic markers may therefore be used in the future as a supplement to clinical information to risk stratify patients with gastric precursors.
Conclusions
NCGA remains a burden to the world, and a leading source of global cancer mortality. Recognizing, detecting, and appropriately surveying precursor lesions (AG, IM, and dysplasia) is an opportunity to intercept NCGA and potentially prevent hundreds of thousands of deaths each year. Tremendous recent advances in endoscopic imaging technology offer the ability for endoscopists to more accurately identify and more precisely classify gastric precursors. Concurrently, publication of several important recent guidelines now places surveillance of gastric precursors within a normative framework for the first time. Integration of emerging technologies such as AI and biomarkers into high-quality clinical practice may further improve our ability to improve early detection of this highly-fatal cancer.
Acknowledgments
The authors would like to thank the Cancer Institute Hospital of the Japanese Foundation for Cancer Research (JFCR), and AI Medical Service Inc. (Tokyo, Japan) for allowing use of endoscopic images for figure creation.
Funding: This work was supported by the National Cancer Institute (No. K08CA252635 to RJ Huang).
Footnote
Provenance and Peer Review: This article was commissioned by the Guest Editor (Terry L. Jue) for the series “A U.S. Perspective on Endoscopic Resection of Neoplastic Lesions of the Gastrointestinal Tract” published in Annals of Laparoscopic and Endoscopic Surgery. The article has undergone external peer review.
Peer Review File: Available at https://ales.amegroups.com/article/view/10.21037/ales-23-13/prf
Conflicts of Interest: All authors have completed the ICMJE disclosure form (available at https://ales.amegroups.com/article/view/10.21037/ales-23-13/coif). The series “A U.S. Perspective on Endoscopic Resection of Neoplastic Lesions of the Gastrointestinal Tract” was commissioned by the editorial office without any funding or sponsorship. JHH and RJH have a sponsored research agreement with AI Medical Service Inc. (Tokyo, Japan). JHH reports consulting fees from Olympus Corporation, Boston Scientific Corporation, Medtronic PLC, and Fujifilm Holdings Corporation. RJH reports permission to use endoscopic images from AI Medical Service Inc. (Tokyo, Japan) and support from the National Cancer Institute under Award Number K08CA252635. The authors have no other conflicts of interest to declare.
Ethical Statement: The authors are accountable for all aspects of the work in ensuring that questions related to the accuracy or integrity of any part of the work are appropriately investigated and resolved. All clinical procedures described in this study were performed in accordance with the ethical standards of the institutional research committee and with the Helsinki Declaration (as revised in 2013). Written informed consent was obtained from the patients for the publication of this article and accompanying images.
Open Access Statement: This is an Open Access article distributed in accordance with the Creative Commons Attribution-NonCommercial-NoDerivs 4.0 International License (CC BY-NC-ND 4.0), which permits the non-commercial replication and distribution of the article with the strict proviso that no changes or edits are made and the original work is properly cited (including links to both the formal publication through the relevant DOI and the license). See: https://creativecommons.org/licenses/by-nc-nd/4.0/.
References
- Morgan E, Arnold M, Camargo MC, et al. The current and future incidence and mortality of gastric cancer in 185 countries, 2020-40: A population-based modelling study. EClinicalMedicine 2022;47:101404. [Crossref] [PubMed]
- Siegel RL, Miller KD, Fuchs HE, et al. Cancer statistics, 2022. CA Cancer J Clin 2022;72:7-33. [Crossref] [PubMed]
- Huang RJ, Koh H, Hwang JH, et al. A Summary of the 2020 Gastric Cancer Summit at Stanford University. Gastroenterology 2020;159:1221-6. [Crossref] [PubMed]
- Rawla P, Barsouk A. Epidemiology of gastric cancer: global trends, risk factors and prevention. Prz Gastroenterol 2019;14:26-38. [Crossref] [PubMed]
- Cheng XJ, Lin JC, Tu SP. Etiology and Prevention of Gastric Cancer. Gastrointest Tumors 2016;3:25-36. [Crossref] [PubMed]
- Ajani JA, D'Amico TA, Bentrem DJ, et al. Gastric Cancer, Version 2.2022, NCCN Clinical Practice Guidelines in Oncology. J Natl Compr Canc Netw 2022;20:167-92. [Crossref] [PubMed]
- Correa P, Piazuelo MB. The gastric precancerous cascade. J Dig Dis 2012;13:2-9. [Crossref] [PubMed]
- Plummer M, Franceschi S, Vignat J, et al. Global burden of gastric cancer attributable to Helicobacter pylori. Int J Cancer 2015;136:487-90. [Crossref] [PubMed]
- Shah SC, Piazuelo MB, Kuipers EJ, et al. AGA Clinical Practice Update on the Diagnosis and Management of Atrophic Gastritis: Expert Review. Gastroenterology 2021;161:1325-1332.e7. [Crossref] [PubMed]
- Nehme F, Rowe K, Palko W, et al. Autoimmune metaplastic atrophic gastritis and association with neuroendocrine tumors of the stomach. Clin J Gastroenterol 2020;13:299-307. [Crossref] [PubMed]
- Rodriguez-Castro KI, Franceschi M, Miraglia C, et al. Autoimmune diseases in autoimmune atrophic gastritis. Acta Biomed 2018;89:100-3. [PubMed]
- Dixon MF, Genta RM, Yardley JH, et al. Classification and grading of gastritis. The updated Sydney System. International Workshop on the Histopathology of Gastritis, Houston 1994. Am J Surg Pathol 1996;20:1161-81. [Crossref] [PubMed]
- Correa P, Piazuelo MB, Wilson KT. Pathology of gastric intestinal metaplasia: clinical implications. Am J Gastroenterol 2010;105:493-8. [Crossref] [PubMed]
- Sung JK. Diagnosis and management of gastric dysplasia. Korean J Intern Med 2016;31:201-9. [Crossref] [PubMed]
- Sauerbruch T, Schreiber MA, Schüssler P, et al. Endoscopy in the diagnosis of gastritis. Diagnostic value of endoscopic criteria in relation to histological diagnosis. Endoscopy 1984;16:101-4. [Crossref] [PubMed]
- Pimentel-Nunes P, Libânio D, Marcos-Pinto R, et al. Management of epithelial precancerous conditions and lesions in the stomach (MAPS II): European Society of Gastrointestinal Endoscopy (ESGE), European Helicobacter and Microbiota Study Group (EHMSG), European Society of Pathology (ESP), and Sociedade Portuguesa de Endoscopia Digestiva (SPED) guideline update 2019. Endoscopy 2019;51:365-88. [Crossref] [PubMed]
- Panteris V, Nikolopoulou S, Lountou A, et al. Diagnostic capabilities of high-definition white light endoscopy for the diagnosis of gastric intestinal metaplasia and correlation with histologic and clinical data. Eur J Gastroenterol Hepatol 2014;26:594-601. [Crossref] [PubMed]
- East JE, Vleugels JL, Roelandt P, et al. Advanced endoscopic imaging: European Society of Gastrointestinal Endoscopy (ESGE) Technology Review. Endoscopy 2016;48:1029-45. [Crossref] [PubMed]
- Magarotto A, Bruno MT, Masci E. Advanced endoscopic imaging: a narrative review. Digestive Medicine Research 2021;4:72. [Crossref]
- Gono K. Narrow Band Imaging: Technology Basis and Research and Development History. Clin Endosc 2015;48:476-80. [Crossref] [PubMed]
- Pimentel-Nunes P, Dinis-Ribeiro M, Soares JB, et al. A multicenter validation of an endoscopic classification with narrow band imaging for gastric precancerous and cancerous lesions. Endoscopy 2012;44:236-46. [Crossref] [PubMed]
- Pimentel-Nunes P, Libânio D, Lage J, et al. A multicenter prospective study of the real-time use of narrow-band imaging in the diagnosis of premalignant gastric conditions and lesions. Endoscopy 2016;48:723-30. [Crossref] [PubMed]
- Uedo N, Ishihara R, Iishi H, et al. A new method of diagnosing gastric intestinal metaplasia: narrow-band imaging with magnifying endoscopy. Endoscopy 2006;38:819-24. [Crossref] [PubMed]
- Buxbaum JL, Hormozdi D, Dinis-Ribeiro M, et al. Narrow-band imaging versus white light versus mapping biopsy for gastric intestinal metaplasia: a prospective blinded trial. Gastrointest Endosc 2017;86:857-65. [Crossref] [PubMed]
- Dilaghi E, Esposito G, Pivetta G, et al. Endoscopic diagnosis of gastric intestinal metaplasia in patients with autoimmune gastritis using narrow-band imaging: does pseudopyloric metaplasia muddy the waters? Endosc Int Open 2022;10:E434-40. [Crossref] [PubMed]
- Esposito G, Pimentel-Nunes P, Angeletti S, et al. Endoscopic grading of gastric intestinal metaplasia (EGGIM): a multicenter validation study. Endoscopy 2019;51:515-21. [Crossref] [PubMed]
- Lee JWJ, Zhu F, Srivastava S, et al. Severity of gastric intestinal metaplasia predicts the risk of gastric cancer: a prospective multicentre cohort study (GCEP). Gut 2022;71:854-63. [Crossref] [PubMed]
- Bi Y, Min M, Zhang F, et al. The Characteristics of Blue Laser Imaging and the Application in Diagnosis of Early Digestive Tract Cancer. Technol Cancer Res Treat 2019;18:1533033819825877. [Crossref] [PubMed]
- Yoshida N, Hisabe T, Hirose R, et al. Improvement in the visibility of colorectal polyps by using blue laser imaging (with video). Gastrointest Endosc 2015;82:542-9. [Crossref] [PubMed]
- Osawa H, Yamamoto H. Present and future status of flexible spectral imaging color enhancement and blue laser imaging technology. Dig Endosc 2014;26:105-15. [Crossref] [PubMed]
- Togashi K, Nemoto D, Utano K, et al. Blue laser imaging endoscopy system for the early detection and characterization of colorectal lesions: a guide for the endoscopist. Therap Adv Gastroenterol 2016;9:50-6. [Crossref] [PubMed]
- Dohi O, Yagi N, Naito Y, et al. Blue laser imaging-bright improves the real-time detection rate of early gastric cancer: a randomized controlled study. Gastrointest Endosc 2019;89:47-57. [Crossref] [PubMed]
- Shinozaki S, Osawa H, Hayashi Y, et al. Linked color imaging for the detection of early gastrointestinal neoplasms. Therap Adv Gastroenterol 2019;12:1756284819885246. [Crossref] [PubMed]
- Osawa H, Miura Y, Takezawa T, et al. Linked Color Imaging and Blue Laser Imaging for Upper Gastrointestinal Screening. Clin Endosc 2018;51:513-26. [Crossref] [PubMed]
- Yasuda T, Yagi N, Omatsu T, et al. Benefits of linked color imaging for recognition of early differentiated-type gastric cancer: in comparison with indigo carmine contrast method and blue laser imaging. Surg Endosc 2021;35:2750-8. [Crossref] [PubMed]
- Ono S, Kato M, Tsuda M, et al. Lavender Color in Linked Color Imaging Enables Noninvasive Detection of Gastric Intestinal Metaplasia. Digestion 2018;98:222-30. [Crossref] [PubMed]
- Fennerty MB. Tissue staining. Gastrointest Endosc Clin N Am 1994;4:297-311. [Crossref] [PubMed]
- Kimura K, Takemoto T. An Endoscopic Recognition of the Atrophic Border and its Significance in Chronic Gastritis. Endoscopy 1969;1:87-97. [Crossref]
- Trivedi PJ, Braden B. Indications, stains and techniques in chromoendoscopy. QJM 2013;106:117-31. [Crossref] [PubMed]
- ASGE Technology Committee. Chromoendoscopy. Gastrointest Endosc 2007;66:639-49. [Crossref] [PubMed]
- Taghavi SA, Membari ME, Eshraghian A, et al. Comparison of chromoendoscopy and conventional endoscopy in the detection of premalignant gastric lesions. Can J Gastroenterol 2009;23:105-8. [Crossref] [PubMed]
- Fennerty MB, Sampliner RE, McGee DL, et al. Intestinal metaplasia of the stomach: identification by a selective mucosal staining technique. Gastrointest Endosc 1992;38:696-8. [Crossref] [PubMed]
- Dinis-Ribeiro M, da Costa-Pereira A, Lopes C, et al. Magnification chromoendoscopy for the diagnosis of gastric intestinal metaplasia and dysplasia. Gastrointest Endosc 2003;57:498-504. [Crossref] [PubMed]
- Sakai Y, Eto R, Kasanuki J, et al. Chromoendoscopy with indigo carmine dye added to acetic acid in the diagnosis of gastric neoplasia: a prospective comparative study. Gastrointest Endosc 2008;68:635-41. [Crossref] [PubMed]
- Lee BE, Kim GH, Park DY, et al. Acetic acid-indigo carmine chromoendoscopy for delineating early gastric cancers: its usefulness according to histological type. BMC Gastroenterol 2010;10:97. [Crossref] [PubMed]
- Song KH, Hwang JA, Kim SM, et al. Acetic acid chromoendoscopy for determining the extent of gastric intestinal metaplasia. Gastrointest Endosc 2017;85:349-56. [Crossref] [PubMed]
- Longcroft-Wheaton G, Duku M, Mead R, et al. Acetic acid spray is an effective tool for the endoscopic detection of neoplasia in patients with Barrett's esophagus. Clin Gastroenterol Hepatol 2010;8:843-7. [Crossref] [PubMed]
- Lambert R, Rey JF, Sankaranarayanan R. Magnification and chromoscopy with the acetic acid test. Endoscopy 2003;35:437-45. [Crossref] [PubMed]
- Iishi H, Tatsuta M, Okuda S. Diagnosis of simultaneous multiple gastric cancers by the endoscopic Congo red--methylene blue test. Endoscopy 1988;20:78-82. [Crossref] [PubMed]
- Phaosawasdi K, Sritunyarat Y, Lopimpisuth C, et al. Prevalence of achlorhydria in an Asian population detected using Congo red staining during routine gastroscopy: 22 years' experience from a single centre. BMJ Open Gastroenterol 2022;9:e000976. [Crossref] [PubMed]
- Shaw D, Blair V, Framp A, et al. Chromoendoscopic surveillance in hereditary diffuse gastric cancer: an alternative to prophylactic gastrectomy? Gut 2005;54:461-8. [Crossref] [PubMed]
- Wasielica-Berger J, Rogalski P, Pryczynicz A, et al. Methylene blue chromoendoscopy is more useful in detection of intestinal metaplasia in the stomach than mucosal pit pattern or vessel evaluation and predicts advanced Operative Link on Gastric Intestinal Metaplasia stages. Clin Endosc 2023;56:203-13. [Crossref] [PubMed]
- Dinis-Ribeiro M. Chromoendoscopy for early diagnosis of gastric cancer. Eur J Gastroenterol Hepatol 2006;18:831-8. [Crossref] [PubMed]
- Polglase AL, McLaren WJ, Skinner SA, et al. A fluorescence confocal endomicroscope for in vivo microscopy of the upper- and the lower-GI tract. Gastrointest Endosc 2005;62:686-95. [Crossref] [PubMed]
- Confocal laser endomicroscopy. Gastrointest Endosc 2014;80:928-38. [Crossref] [PubMed]
- Meyer RR, Simpson MV. DNA biosynthesis in mitochondria. Differential inhibition of mitochondrial and nuclear DNA polymerases by the mutagenic dyes ethidium bromide and acriflavin. Biochem Biophys Res Commun 1969;34:238-44. [Crossref] [PubMed]
- Wallace MB, Meining A, Canto MI, et al. The safety of intravenous fluorescein for confocal laser endomicroscopy in the gastrointestinal tract. Aliment Pharmacol Ther 2010;31:548-52. [Crossref] [PubMed]
- Pilonis ND, Januszewicz W, di Pietro M. Confocal laser endomicroscopy in gastro-intestinal endoscopy: technical aspects and clinical applications. Transl Gastroenterol Hepatol 2022;7:7. [Crossref] [PubMed]
- Guo YT, Li YQ, Yu T, et al. Diagnosis of gastric intestinal metaplasia with confocal laser endomicroscopy in vivo: a prospective study. Endoscopy 2008;40:547-53. [Crossref] [PubMed]
- Wang TD. Confocal microscopy from the bench to the bedside. Gastrointest Endosc 2005;62:696-7. [Crossref] [PubMed]
- Fugazza A, Gaiani F, Carra MC, et al. Confocal Laser Endomicroscopy in Gastrointestinal and Pancreatobiliary Diseases: A Systematic Review and Meta-Analysis. Biomed Res Int 2016;2016:4638683. [Crossref] [PubMed]
- Huang RJ, Choi AY, Truong CD, et al. Diagnosis and Management of Gastric Intestinal Metaplasia: Current Status and Future Directions. Gut Liver 2019;13:596-603. [Crossref] [PubMed]
- Pittayanon R, Rerknimitr R, Wisedopas N, et al. The learning curve of gastric intestinal metaplasia interpretation on the images obtained by probe-based confocal laser endomicroscopy. Diagn Ther Endosc 2012;2012:278045. [Crossref] [PubMed]
- Lim LG, Yeoh KG, Srivastava S, et al. Comparison of probe-based confocal endomicroscopy with virtual chromoendoscopy and white-light endoscopy for diagnosis of gastric intestinal metaplasia. Surg Endosc 2013;27:4649-55. [Crossref] [PubMed]
- Zhang HP, Yang S, Chen WH, et al. The diagnostic value of confocal laser endomicroscopy for gastric cancer and precancerous lesions among Asian population: a system review and meta-analysis. Scand J Gastroenterol 2017;52:382-8. [Crossref] [PubMed]
- ASGE Technology Committee. Autofluorescence imaging. Gastrointest Endosc 2011;73:647-50. [Crossref] [PubMed]
- Haringsma J, Tytgat GN. Fluorescence and autofluorescence. Baillieres Best Pract Res Clin Gastroenterol 1999;13:1-10. [Crossref] [PubMed]
- Ragunath K. Autofluorescence endoscopy--not much gain after all? Endoscopy 2007;39:1021-2. [Crossref] [PubMed]
- Kato M, Kaise M, Yonezawa J, et al. Autofluorescence endoscopy versus conventional white light endoscopy for the detection of superficial gastric neoplasia: a prospective comparative study. Endoscopy 2007;39:937-41. [Crossref] [PubMed]
- Yan T, Wong PK, Choi IC, et al. Intelligent diagnosis of gastric intestinal metaplasia based on convolutional neural network and limited number of endoscopic images. Comput Biol Med 2020;126:104026. [Crossref] [PubMed]
- Zhang Y, Li F, Yuan F, et al. Diagnosing chronic atrophic gastritis by gastroscopy using artificial intelligence. Dig Liver Dis 2020;52:566-72. [Crossref] [PubMed]
- Hirasawa T, Aoyama K, Tanimoto T, et al. Application of artificial intelligence using a convolutional neural network for detecting gastric cancer in endoscopic images. Gastric Cancer 2018;21:653-60. [Crossref] [PubMed]
- Nagao S, Tsuji Y, Sakaguchi Y, et al. Highly accurate artificial intelligence systems to predict the invasion depth of gastric cancer: efficacy of conventional white-light imaging, nonmagnifying narrow-band imaging, and indigo-carmine dye contrast imaging. Gastrointest Endosc 2020;92:866-873.e1. [Crossref] [PubMed]
- Gupta S, Li D, El Serag HB, et al. AGA Clinical Practice Guidelines on Management of Gastric Intestinal Metaplasia. Gastroenterology 2020;158:693-702. [Crossref] [PubMed]
- Dinis-Ribeiro M, Areia M, de Vries AC, et al. Management of precancerous conditions and lesions in the stomach (MAPS): guideline from the European Society of Gastrointestinal Endoscopy (ESGE), European Helicobacter Study Group (EHSG), European Society of Pathology (ESP), and the Sociedade Portuguesa de Endoscopia Digestiva (SPED). Endoscopy 2012;44:74-94. [Crossref] [PubMed]
- Banks M, Graham D, Jansen M, et al. British Society of Gastroenterology guidelines on the diagnosis and management of patients at risk of gastric adenocarcinoma. Gut 2019;68:1545-75. [Crossref] [PubMed]
- Lahner E, Zagari RM, Zullo A, et al. Chronic atrophic gastritis: Natural history, diagnosis and therapeutic management. A position paper by the Italian Society of Hospital Gastroenterologists and Digestive Endoscopists [AIGO], the Italian Society of Digestive Endoscopy [SIED], the Italian Society of Gastroenterology [SIGE], and the Italian Society of Internal Medicine Dig Liver Dis 2019;51:1621-32. [SIMI]. [Crossref] [PubMed]
- ASGE Standards of Practice Committee. The role of endoscopy in the management of premalignant and malignant conditions of the stomach. Gastrointest Endosc 2015;82:1-8. [Crossref] [PubMed]
- Nieuwenburg SAV, Mommersteeg MC, Eikenboom EL, et al. Factors associated with the progression of gastric intestinal metaplasia: a multicenter, prospective cohort study. Endosc Int Open 2021;9:E297-305. [Crossref] [PubMed]
- Huang KK, Ramnarayanan K, Zhu F, et al. Genomic and Epigenomic Profiling of High-Risk Intestinal Metaplasia Reveals Molecular Determinants of Progression to Gastric Cancer. Cancer Cell 2018;33:137-150.e5. [Crossref] [PubMed]