Mesh technology—an update
Introduction
Use of prosthesis has revolutionized the results of abdominal wall hernia repair. Surgical prosthesis today is an indispensable tool in the surgeon’s armamentarium for management of abdominal wall hernia. For the purpose of this review, the term abdominal wall hernia will include ventral abdominal and incisional hernia. Repair of abdominal wall hernia is a commonly performed surgical procedure worldwide. Except in very small defects, mesh repair is considered gold standard and universally accepted for management of abdominal wall hernia. To improve outcomes of surgical repair, over years surgeons have evolved the surgical technique ranging from the approach used, to the plane in which a mesh is placed in the anterior abdominal wall and the peritoneum. With reasonable standardization of techniques, the focus has now shifted towards improvement of mesh technology. New meshes continue to be developed and the surgeon today has to address the dilemma of selecting an appropriate prosthetic material and variety of designs from an array of manufacturers.
History of mesh use in abdominal wall hernia repair
Over years researchers have worked relentlessly to improve the understanding of the exact causes of hernia formation and its biological basis. Our knowledge has evolved and improved over last 6–7 decades. Armed with this knowledge, most researchers have evolved the techniques of hernia repair and advocated the use of mesh to manage abdominal wall hernias. The first hernia prosthetics were made from metal. In 1894, Phelps demonstrated use of silver wire braided meshes. In 1900, Goepel, Perry and Witzel too used these meshes for hernia repair (1-4). The metal component produced stiffness and the prosthesis was soon out of favour. Even worse, few reports also suggested formation of silver sulfate over its surface which is toxic by nature. This resulted in modification of the meshes, ultimately being replaced with braided stainless steel meshes (5-7). The search for better materials continued. Starting 1948 onwards in the next 15–20 years various prostheses incorporated the use of tantalum gauze, perlon meshes or prefabricated nylon (8-13). None of these meshes however, were without problems. While Nylon was reported to break apart, tantalum gauze reported higher rates of infection, and perlon was associated with an intense inflammatory reaction, ultimately all were abandoned.
With dawn of 20th century, use of newer biomaterials like nylon mesh, polyvinyl sponges, orlon cloth, teflon and silicon meshes was reported. But were soon abandoned due to complications or sub-optimal results (14). The start of 2nd world war lead to increased demand of steel and tantalum for use in military equipment, raising the cost and scarcity. Simultaneously there was rise of plastic and polymer industry. The combined effect was that surgeons and fabricators, were compelled to develop new prosthesis based on polymers. Materials like polypropylene, the expanded polytetrafluoroethylene (ePTFE) and polyester were the result of this shift from metal-based prosthesis to polymers. These three materials are the most commonly used in various meshes available today (13,15,16).
Basis for use of mesh for managing abdominal wall hernia
The main principle in repair of abdominal wall hernia is restoration of normal anatomy without any undue tension, following the fundamentals of “tension free repair”. The main goal in any abdominal wall hernia repair is to avoid or minimise recurrences. Any mesh works as a scaffold over which new tissue grows, providing mechanical stability and strength to the muscle and fascial tissues. An understanding of anatomy of abdominal wall, factors leading to hernia formation, pathophysiology of wound healing and the tissue response to the inserted prosthesis is necessary to fully understand the importance a prosthesis plays in hernia repair and its long-term results.
Biological response to mesh
The understanding of wound healing, a complex and dynamic process, is necessary for surgeons before performing hernia repair surgeries. The healing process involves multiple complex and sequential events. After mesh implantation, protein adsorption around the prosthesis is the first process to start, ultimately forming a coagulum. Albumin, immunoglobulins, plasminogen, fibrinogen, and complement factors combine together to form the coagulum (17,18), which in turn causes platelet adherence. The adhered platelets by releasing chemo-attractants, attracts polymorphonucleocytes (PMNs), fibroblasts, macrophages as well as other platelets. Coagulum formation as well as chemoattraction process is influenced by a variety of factors. These include the material used in prosthesis, its design, presence or absence of infection, medicines and certain hitherto unknown events. This is followed by a foreign body reaction which is intense and in turn leads to deposition of collagen in extracellular matrix. Over a period of time the collagen transformation takes place replacing immature collagen by mature form. End result being increasing the collagen strength. By 6 months, the strength of collagen increases gradually but never regain complete strength of original native connective tissue, reaching about 70–80% (19,20). This lack of complete regain of strength justifies the use of prosthetic mesh to provide a permanent and long-lasting support.
Classification of meshes
Multiple factors decide which type of mesh or prosthesis a surgeon uses. Meshes are classified based on the type of material, prosthesis design and coatings used if any to cover the mesh material. They can be classified into synthetic and biologic broadly, based upon the type of material and its associated biological behaviour. Further based upon the degradation and tissue reaction synthetic mesh are further classified into partially absorbable, non-absorbable and non-absorbable coated meshes.
In 1997, Amid (21) classified biomaterials into four types depending upon mesh porosity into:
- Totally macroporous mesh: prosthesis with pore size larger than 75 micrometer e.g., Prolene, Marlex;
- Completely microporous mesh: prosthesis with pore size <10 micrometer e.g., ePTFE;
- Macroporous mesh with microporous and multi-filamentous components e.g., braided polypropylene or dacron mesh;
- Prosthesis with submicronic pores e.g., silastic.
With advent of multiple new materials in market, changes in designing of mesh as well the fibres, the concept of mesh porosity lost favour with researchers. Mesh density and weight were considered to be more important parameters, and Coda et al. (22) proposed a classification taking into consideration these two factors.
- Heavy weight mesh ≥140 gram/m2;
- Standard weight mesh 70–140 gram/m2;
- Light weight mesh 35–70 gram/m2;
- Ultralight weight mesh ≤35 gram/m2.
The tissue reaction to the mesh material used involves a complex number of biological and host response. Apart from the material used it is also affected by the architecture of mesh, mesh design. Factors such a film, fleece and filament structure also play a vital role. Less heavy weight mesh does not essentially mean lesser biological and host response, this was proved in 2006 by Weyhe et al. when they compared light weight and heavy prosthesis (23). In fact, some meshes in the heavy weight category demonstrate a very good tissue biocompatibility. The reason being the large pores which reduces process of scar bridging. Reduction in bridging of scar has been proven to be associated with reduction in mesh contraction (24,25). Over the years with improved knowledge of the mesh induced biological response, we clearly know that multi-factorial interaction takes place which modifies the biological and tissue response to mesh, which also affects the host compatibility. With rising popularity of intra-abdominal mesh placement due to laparoscopy, Deeken et al. felt the need to further sub-classify the meshes that have barrier function additionally in 2012 (26). Attempts were made to classify prosthesis using their properties such as elasticity and biomechanical stability. This classification system however failed to gain popularity due to marked anisotropy, as different values were seen in different axis (27-30).
Klinge et al. in the same year made an attempt to overcome limitations of anisotrophy (31), categorized and subclassified meshes into classes, taking the textile data of the mesh into consideration and suggested the concept “mesh porosity”. To nullify the effect of anisotropy, they suggested “effective porosity” to classify meshes as:
- Class 1 mesh—large pore (>60% textile porosity or >0% effective porosity). Large pored meshes were additionally sub-classified as:
- Monofilament mesh;
- Multifilament mesh;
- Mesh with mixed structure or combination of multiple polymer i.e., combination of different non-absorbable materials or a combined non-absorbable and absorbable polymer.
- Class 2 mesh—small pore (no effective porosity but <60% textile porosity). These meshes too were sub-classified as:
- Monofilament mesh;
- Multifilament mesh;
- Meshes with mixed structure or combination of polymers.
- Class 3 Mesh—having special type of features e.g., meshes with special surface coatings, composite meshes or covered meshes generally developed for intra-abdominal usage.
- Class 4 Mesh—with films i.e., meshes with submicronic pores or without any porosity.
- Class 5 Mesh—3D i.e., meshes or devices which are pre-shaped, different from the routine flat mesh.
- Class 6 Mesh—biological prosthesis: these were further sub-grouped as non-absorbable or absorbable, as well as synthetic or biological depending on the source. These were then sub-divided as:
- Meshes which are cross linked;
- Meshes which are Non-cross linked;
- Meshes having special feature.
Mesh material
An understanding of different raw materials is necessary before deciding which mesh to be used. Different clinical scenarios may necessitate use of different raw materials. Most surgeons will consider the ease of mesh handling, more so during laparoscopic mesh repair. Mesh handling characteristics may have a bearing over the operative time as well as results. For ease of understanding we can divide the meshes into:
Non-absorbable synthetic meshes
Most frequently used mesh for repair of ventral hernia are polypropylene, polytetrafluoroethylene (PTFE) or polyester based. Different polymers will induce a variable tissue response (32).
Polypropylene: an Italian researcher Giolo Natta, developed this material in early 1950’s. A polymerized ethylene, it is the most commonly used polymer in hernia repair surgery. During the process of polymerization, the position where the methyl component attaches on ethylene decides the strength the prosthesis achieves. If all of these methyl components attach to one side of the ethylene, the maximum strength is achieved (33). Polypropylene by its hydrophobic nature, is electrostatically neutral—demonstrating inherit resistance to the process of degradation by host of tissue responses. Surgeons generally prefer use of more porous meshes, in order to minimise the inflammatory reaction, which in turn also prevents bridging of scar (34,35). The debate regarding an ideal prosthesis is still not settled, with individual preferences dictating the choice of mesh. Polypropylene by inducing an intense biological response, causes protein adherence, which in turn leads to scar tissue formation. Amount of scarring will decide the level of mesh contraction. The same protein adherence, forms adhesions at the mesh abdominal wall interface. One of the common causes for chronic pain or operative site discomfort post hernia repair surgery is intense scarring and adhesion (36,37). If used intra-abdominally, the mesh comes in contact with the contents e.g., bladder/bowel to form similar adhesions. At times this may lead to intestinal obstruction or fistulisation, which are challenging scenario to manage in addition to the patient morbidity that follows. This led to development of prosthesis having a barrier or composite mesh which can be used inside the peritoneal cavity. Although how an individual host tissue responds to the prosthesis, plays a pivotal role in adhesion and scarring (38-41).
Polyester: polyester is a common name used for polyethylene terephthalate, made up of terephthalic acid and ethylene glycol. Due to its hydrophilic nature, it swells up on contact with tissue fluids. The biologic response induced though similar to polypropylene, causes polyester to degrade over long period of time. In an infective environment, the degradation is accentuated (42).
Polytetrafluoroethylene (PTFE): born out of an accidental discovery in 1938 while working on a gas refrigerant—tetrafluoroethylene, Roy Plunkett documented that the refrigerant gas polymerizes spontaneously to form a wax like powdery material. Twenty years later in 1958, William Gore used the same process to manufacture PTFE based meshes. With poor tissue incorporation and its micro-porous nature which prevents passage of protein coagulum, PTFE causes encapsulation by the scar tissue. The micropores though allow bacteria to pass and form a biofilm making it difficult to manage if infected. This forms the basis why if infected, PTFE based prosthesis invariably need to be removed out. To overcome this, researchers manufacture PTFE in an expanded or condensed form in order to achieve uniformity in structure. Hence the names ePTFE and cPTFE respectively.
Other polymers which have been used are polyvinylidenflouride or fibres made from carbon. These materials have never been popular with surgeons.
Non-absorbable synthetic coated mesh
To reduce protein adherence and in turn the scar formation and inflammatory reaction, researchers manufactured prosthesis with an absorbable or non-absorbable coating over the primary polymer namely polypropylene and polyester scaffold (43,44). Reduced scarring and adhesions was though to reduce mesh shrinkage as well as the incidence of chronic pain. Less mesh shrinkage also meant better overlap and possibly reduced recurrence rates (44). A variety of coating materials were manufactured e.g., titanium, PVDF coating, coating of Omega-3 fatty acid (O-3FA), hyaluronate based, all with their own drawbacks and complications (45,46).
- Titanized polypropylene meshes: two titanium coated meshes available are TiO2 Mesh (Biocer, Germany), TiMesh (GFE Medizintechnik, Germany). In Timesh, the filament made from polypropylene is coated with layer of titanium, 30 micron-meter thick by the deposition technique where plasma is used for activating chemical vapor. Titanium by improving biocompatibility reduces adhesions. Based on filament tensile strength and filament size, the mesh is manufactured as strong (120 micron-meter), light (90 micron-meter) and extralight (65 micron-meter). The filament of polypropylene is covered by titanium dioxide in TiO2 mesh. Titanium dioxide by its hydrophilic nature is thought to produce a self-adhesive action. Manufactured as a mesh with large pores, providing around 55 N/cm of tensile strength.
- ePTFE meshes are manufactured as a two-sided DualMesh, itRamesh T1, Compasix mesh and Dulex. The DualMesh is coated with silver-chlorhexidine film, providing an anti-microbial effect.
- ParieteneTM DS Composite mesh (Medtronic, USA) is a macroporous mesh made up of woven 2-D polypropylene which is covered by an oxidized collagen film on one surface. An absorbable polycaprolactone is used as a binding agent and markings for orientation of mesh. The collagen film by reducing adhesions protects the viscera. SymbotexTM Composite mesh (Medtronic, USA), uses a 3D textile monofilament polyester coated on one side with a bioabsorbable collagen film.
- VentralightTM mesh (Bard, Warwick, UK) made from polypropylene, makes use of hydrogel barrier along with a bioreabsorbable cover made up of polyglycolic acid.
- Other coated prosthesis is C-QURTM Centrifix mesh and C-QurTM (Atrium medical) and is an O-3FA coated filament polypropylene mesh. Glucamesh (Brennen Medical, Minnesota) a polypropylene based prosthesis, has an oat beta glucan coating, Dynamesh (FEG Textiltechnik, Germany) again a polypropylene based mesh which is coated by PVDF filament, but mostly tried in inguinal hernia repair.
Partially absorbable meshes
With the aim to minimize the total amount of foreign material, ultimately leading to lesser tissue inflammatory reaction, clinical researchers conceptualised partially absorbable meshes. These were made up of a combination of absorbable as well as non-absorbable components. Other possible advantages suggested were lighter mesh and large pore size post absorbable component degradation. But till date no conclusive evidence both in animal as well as human studies, have demonstrated clinical superiority of these meshes over standard non-absorbable meshes, both in terms of biological and inflammatory response induced and clinical outcomes namely recurrence (46-48).
Compared to a heavy mesh, theoretical advantages proposed are large pore, light weight, lesser inflammatory response, better flexibility and elasticity with resultant less shrinkage and possible less pain and infection (49). But maintaining the tensile strength of the mesh after degradation of absorbable component is a challenge. To achieve an ideal balance between the mesh weight and density, its resultant porosity ultimately culminating into the prosthesis tensile strength after partial resorption is not an easy task. Limited research has still demonstrated complications like adhesion formation, mesh contraction, infection and recurrences (45). Most clinical researches on these meshes have been in groin hernias and more evidence is needed before advocating use of these meshes in ventral hernia repair and reaching a definitive conclusion.
Biological mesh
Occasionally a surgeon is challenged with clinical scenarios where a hernia gets strangulated, obstructed and when there is tissue contamination e.g., bowel injuries with spillage, presence of infection. To overcome these challenge researchers developed biological meshes. Made mainly from collagen, these are supposed to help native cells and connective tissue to bridge by providing a scaffold and cover the hernial defect. Various tissues such as bovine pericardium, bovine or porcine dermis, porcine small bowel submucosa and decellularized human dermis are used. Most of these materials will get absorbed within 3–4 months. In order to improve the duration that the mesh persists, these are subjected to process of chemical crosslinking during commercial production. Based upon this process, some biological meshes take up to 12 months to get completely re-absorbed. As we all known in the process of wound healing, the new connective tissue matrix can only regain about 70–80% strength of native connective tissue. Therefore, technically complete resorption will cause higher recurrences. Another major issue is cost, limiting its wide spread use. Hence routine use is advocated only during special clinical scenarios and in investigational studies.
- Surgisis: is a mesh developed from submucosa of porcine small intestine (Cook Biomedical, USA).
- Permacol: in this mesh porcine dermis is used after processing it by diisocyanate, and then sterilization with gamma-irradiation (Covidien, CT).
- XenMatrix: manufactured too from porcine dermis but non-crosslinked, it used E-beam radiation for sterilization (Brennen Medical, MN).
- Collamend: here the porcine dermis is subjected to freeze drying process (Davol Inc., RI).
- Tutopatch: developed from the bovine pericardium. The pericardium, then undergoes a series of processing by chemicals. Finally sterilized with gamma-irradiation (Tutogen, FL).
- Veritas (Synovis Surgical innovations, St. Paul, MN) is similarly made up from pericardial layers of young or non-adult cow.
- Cadaveric allograft: commercially marketed, almost all undergo some mode of processing before commercial use.
- Alloderm—developed from cadaveric skin, which undergoes a variety of processing techniques, taking due precautions to avoid tissue injury or damage to the layer of extra-cellular matrix (LifeCell Corporation, NJ). Any remnant cells are removed using deoxycholate. This is further processed by cleaning and lyophilization. The end product is a sheet of extracellular matrix made up of collagen, elastin and laminin, which is then preserved by freeze drying. During clinical use we need to soak it in saline to defreeze.
- Allomax (Tutogen Medical Inc., Alachua, FL) which is marketed by Davol, uses human dermis which is processed to produce an acellular sheet. This sheet like any other mesh acts as a scaffold.
- Researchers have also used scaffold made up from musculoskeletal tissue. Use of various growth factors e.g., fibroblast growth factors to stimulate collagen synthesis has been attempted in animal studies.
Mesh with special design/structure
Search for mesh which fulfils all ideal criteria continues. Over years with advent in minimal invasive surgery, laparoscopic repair of ventral/incisional hernia gained popularity. Researchers and manufactured hence focused their attention to designing meshes which were compatible with laparoscopic repair techniques. Laparoscopic mesh handling, ease of mesh positioning, meshes with prefixed sutures, composite meshes, meshes which minimize or remove the necessity for use of fixation device, meshes which could be inserted through laparoscopic trocars were designed.
Meshes with special design
With the intent to help mesh handling and positioning meshes with variety of shapes and design are manufactured for use in laparoscopy. As most meshes are placed intra-abdominally by intraperitoneal onlay method (IPOM), where mesh is exposed to the visceral structures various composite meshes were designed. VentralightTM ST mesh with an ECHO PSTM positioning system (Bard, Davol Inc., Warwick, UK) is a polypropylene mesh covered on the visceral surface with a hydrogel barrier and bioreabsorbable polyglycolic acid. The ECHO positioning system is a deployment and positioning device which comes attached to the mesh (Figure 1). It is claimed that this facilitates mesh positioning and centering over the hernia defect particularly when large meshes are used. The entire system consists of a balloon attached to the mesh visceral surface, connectors, inflation tube and syringe. There is a special introducer for inserting the mesh through trocars. ComposixTM L/P mesh (Bard, Davol Inc., Warwick, UK), a low-profile large pore polypropylene/ePTFE mesh also comes with attached ECHO positioning system. Once mesh is positioned and fixed, the entire positioning system is removed after deflating the balloon.
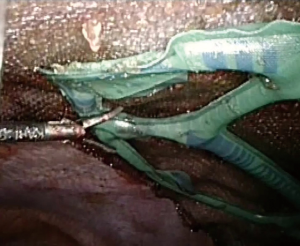
For smaller ventral hernias particularly umbilical, para-umbilical, epigastric and trocar site hernia special devices which could be inserted through a small incision were devised. Here the mesh is placed in an IPOM technique during open hernia repair. VentralexTM ST hernia patch (Bard, Davol Inc., Warwick, UK) comes with SorbaflexTM memory technology and an absorbable barrier using the sepra technology. Similarly, the ParietexTM composite ventral patch (Medtronic, Trevoux, France) is a dual-facing non-absorbable mesh (Figure 2). It is made up of 3-D monofilament polyester textile for abdominal wall reinforcement. The visceral surface is covered with bioabsorbable hydrophilic collagen film. The design includes an absorbable expander for facilitating placement, fixation system with four flaps and two removable handles. The ProceedTM ventral patch (Ethicon, USA), made up of soft polypropylene, the visceral surface is covered with oxidized regenerated cellulose fabric and that in contact with abdominal wall has polyglactin with side straps to facilitate placement and fixation. All these meshes are available in different sizes, and ideally meant for use in ventral hernia defects generally smaller than 3 cm.
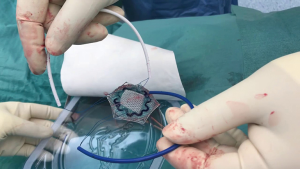
With the goal of reducing fixation associated postoperative pain and possibly operative times, mesh designs which do not need fixation were devised. The OctomeshTM freedom ventral hernia repair system (Insightra, USA), is polypropylene mesh designed for retro-rectus or preperitoneal mesh placement in open ventral hernia repair. It consists of 8 anchoring straps which are tunnelled through the abdominal wall and the friction produced is used to stabilize the mesh. It comes with a strap passer to tunnel the anchoring straps. Parietex ProGripTM self-fixating mesh (Medtronic, Trevoux, France), is made up of hydrophilic monofilament polyester with a resorbable polylactic acid (PLA) microgrip technology. By avoiding need for fixation devices or transfascial sutures, they should theoretically reduce pain and operative time. These can be used both in open ventral/incisional or in laparoscopic repair for pre-peritoneal and retro-rectus mesh placement.
The knitting technology
During manufacturing meshes, the fibres are curved so as to follow a meandering path. This is different from the universal oriented technique used for manufacturing non-medical use nets or clothes i.e., weaving. The fibres itself can be monofilament or multifilament. Based upon the knitting of fibres, the warp knits and weft knits can be generated. The end result is a soft, flexible and stretchable structure which easily adapts to the contour of human body during movements (30). It also provides increased tensile strength, bursting strength and good porosity. The goal is to try and mimic the abdominal wall biomechanical characteristics i.e., tension of 16 N/cm with an elasticity of roughly 38% (45). The process of interweaving and warp knitting of the primary material prevents loosening or peeling of meshes when cut to refashion or shape a mesh during insertion or in surgeries wherein we may need to cut through old meshes e.g., laparotomy in patients with previous hernia mesh repair. The integrity of mesh is preserved regardless of the direction in which it is cut (50).
Prosthesis with emphasis on cost
The burden of untreated hernias is huge in underdeveloped and low-income nations. To add to this, it also parallels poor access to quality surgical care. Hence mesh cost is an important factor causing significant impact on medical resources and healthcare for low income nations. Manufactures are taking all efforts, so as to create a cost effective and cheap alternative, which is simple to produce, ease of availability, safety and feasibility for mass manufacturing. Sterilized mosquito nets use has been documented in repair of groin hernia from India and Africa, with clinically acceptable and safe outcomes. In experimental studies also there, tensile strength and characteristics have been found comparable to standard polypropylene meshes (51-56). There are also trials ongoing to test the efficacy of these nets for ventral hernia repair (57). Most of these nets are commonly made up of Nylon. A polyethylene based low-priced prosthesis is marketed by Amsa Plastics from Karur in India.
Hernia, prosthesis and its technology—research focus in future
Any new research in future on hernia invariably will be focused on development of never mesh technologies and modification of operative techniques with emphasis of improved clinical results and reduced complications. Way back in 1878, Billroth T. once told his student Czerny, “If we can artificially produce tissues of the density and toughness of fascia and tendon the secret of the radical cure of hernia would be discovered.”
Though exact mechanisms through which a hernia occurs is debatable, it is the result of an interaction and disparity between the resistance offered by musculature of abdominal wall to the outward pressure exerted by the intra-abdominal contents. An interplay of multiple biological processes takes place, which ultimately lead to failure of the surgical wound achieved through changes in the fascial and tissue pathology.
Literature review now proves that various systemic and genetic level interactions, its associated disorders, in turn leads to changes in the quality and structure of connective tissue and the extracellular matrix. This change ultimately leads to abnormalities predisposing an individual to development of a hernia. We can safely state that while a primary hernia occurs by congenital deficiencies in extracellular and tissue matrix. While in an incisional hernia or recurrent hernias, acquired deficiencies in the extracellular and tissue matrix are involved e.g. failed laparotomy wound. Acquired collagen defect also occur in patients who are smokers, obese, have nutritional deficiencies e.g., vitamin deficiencies, copper and zinc deficiencies, disorder of lathyrism. Similarly, factors altering the tissue perfusion and oxygenation i.e., tissue hypoxia is also a risk factor for hernia development as well as recurrence.
Understanding this complex interplay of various factors and the underlying mechanism is important key to reduce recurrence rates and provide optimal results after abdominal wall hernia repair. Hence future research needs to focus more on understanding the biology of extracellular and tissue matrix. The goal is to optimize hernia repair techniques and surgical outcomes. Research in future should target and be focused on altering these very factors and development of newer meshes minimally affecting the complex interplay of tissue reactions leading to tissue matrix deficiencies. Also, newer meshes which can augment the quality of synthesized extra-cellular and tissue matrix can be a topic of interest for researchers.
Factors which should influence mesh choice
Whether prosthesis will ever incorporate all the properties of an ideal mesh is not known. We are still a long way from that goal. As a surgeon, using an appropriate mesh is important, and this choice may vary from patient to patient, but we need to consider certain factors before planning to use any mesh for hernia repair (58).
- Handling ease—of particular importance in minimal invasive techniques.
- Mesh tensile strength—all mesh should have a tensile strength sufficient to withstand a force of around 16 N/cm, in order to reduce disruptions.
- Reduced scar bridging—large pore size ranging between 600–800 micron-meter avoids scar bridging as well as mesh contraction.
- Mesh density—although a definitive consensus is lacking, it should be in the range of 28–90 gram/m2.
- Polymer architecture—knitting technique, mono-filament or braided, woven or solid.
- Based on patient presentation and features—elective setting or emergency procedure, presence/suspicion of infection, defect size, loss of domain.
- Planned Mesh placement—position where mesh is planned to be placed, intra or extra peritoneal, or in between the various layer anterior abdomen musculature.
- Cost.
Conclusions
The search for an ideal mesh will never end. Hence it is paramount that the goal of this search should be to maximize patient benefit. Researchers should focus attention on development of prosthesis which will withstand disruptive force, minimise contraction, be durable and compatible and also produce a suitable biological response. The search needs to be fuelled with adequate funding to researchers. Researchers should have access to laboratory with animal models, so that prosthesis can be tested on tissues which may mimic human body. No animal study will ever recreate human tissue completely, hence clinical research is mandatory. Also make sure sufficient research and patient safety protocols are in place namely, ethical/institutional research and review boards. Adequate audits both at hospital and government level agencies are conducted so as to avoid violation of patient safety and care. Patient counselling is paramount before use of any new prosthesis. Any good mesh if positioned wrongly, without adequate overlap, under tension and without suitable fixation will invariably cause recurrence as well as postoperative complication. Hence surgical skill development and following standard principles of hernia repair cannot be more emphasized.
Acknowledgments
Funding: None.
Footnote
Provenance and Peer Review: This article was commissioned by the editorial office, Annals of Laparoscopic and Endoscopic Surgery for the series “Ventral Hernia”. The article has undergone external peer review.
Conflicts of Interest: Both authors have completed the ICMJE uniform disclosure form (available at http://dx.doi.org/10.21037/ales.2018.09.10). The series “Ventral Hernia” was commissioned by the editorial office without any funding or sponsorship. DL served as the unpaid Guest Editor of the series. The authors have no other conflicts of interest to declare.
Ethical Statement: The authors are accountable for all aspects of the work in ensuring that questions related to the accuracy or integrity of any part of the work are appropriately investigated and resolved.
Open Access Statement: This is an Open Access article distributed in accordance with the Creative Commons Attribution-NonCommercial-NoDerivs 4.0 International License (CC BY-NC-ND 4.0), which permits the non-commercial replication and distribution of the article with the strict proviso that no changes or edits are made and the original work is properly cited (including links to both the formal publication through the relevant DOI and the license). See: https://creativecommons.org/licenses/by-nc-nd/4.0/.
References
- Goepel R. Uber die Verschliessung von Bruchpforten durch Einheilung geflochtener fertiger Silberdrahtnetze. Zentralbl Chir 1900;17:3.
- Perry HB. Implantations of silver filigree for cure of large ventral hernia; report of two cases. Boston Med Surg J 1904;151:2. [Crossref]
- Witzel O. Uber den Verschluss van Bauchwunden und Bruchpforten durch versenkte Silberdrahtnetze. Zetralbl Chir 1900;27:3.
- Phelps A. A new operation for hernia. NY Med J 1894;60:291.
- Preston DJ, Richards CF. Use of wire mesh prostheses in the treatment of hernia. 24 years’ experience. Surg Clin. North Am 1973;53:549-54. [Crossref] [PubMed]
- Mathieson AJ, James JH. A review of inguinal hernia repair using stainless steel mesh. J R Coll Surg Edinb 1975;20:58-62. [PubMed]
- Thomeret G, Dubost C, Pillot P. The use of inoxydizable steel gauze in the treatment of eventrations of hernias. Mem Acad Chir (Paris) 1960;86:500-7. [PubMed]
- Douglas DM. Repair of large herniae with tantalum gauze; an experimental and clinical study. Lancet 1948;1:936-9. [Crossref] [PubMed]
- Throckmorton TD. Tantalum gauze in the repair of hernias complicated by tissue deficiency; a preliminary report. Surgery 1948;23:32-46. [PubMed]
- Koontz AR, Kimberly RC. Tantalum and marlex mesh (with a note on marlex thread): an experimental and clinical comparison - preliminary report. Ann Surg 1960;151:796-804. [Crossref] [PubMed]
- Cumberland VH. A preliminary report on the use of prefabricated nylon weave in the repair of ventral hernia. Med J Aust 1952;1:143-4. [PubMed]
- Doran FS, Gibbins RE, Whitehead R. A report on 313 inguinal herniae repaired with nylon nets. Br J Surg 1961;48:430-4. [Crossref] [PubMed]
- Usher FC, Wallace SA. Tissue reaction to plastics; a comparison of nylon, orlon, Dacron, Teflon, and Marlex. AMA Arch Surg 1958;76:997-9. [Crossref] [PubMed]
- DeBord JR. The historical development of prosthetics in hernia surgery. Surg Clin North Am 1998;78:973-1006. [Crossref] [PubMed]
- Usher FC, Ochsner J, Tuttle LL. Jr. Use of marlex mesh in the repair of incisional hernias. Am Surg 1958;24:969-74. [PubMed]
- Adloff M, Arnaud JP. Surgical management of large incisional hernias by an intraperitoneal Mersilene mesh and an aponeurotic graft. Surg Gynecol Obstet 1987;165:204-6. [PubMed]
- Tang L, Ugarova TP, Plow EF, et al. Molecular determinates of acute inflammatory response to biomaterials. J Clin Invest 1996;97:1329-34. [Crossref] [PubMed]
- Busuttil SJ, Ploplis VA, Castellino FJ, et al. A central role for plasminogen in the inflammatory response to biomaterials. J Thromb Haemost 2004;2:1798-805. [Crossref] [PubMed]
- Woloson SK, Greisler HP. Biochemistry, immunology, and tissue response to prosthetic material.in: Bendavid et al, (Ed.) Abdominal wall hernias, principles and management. New York: Springer-Verlag, 2001;201-7.
- Diegelmann RF. Collagen metabolism. Wounds 2000;13:177-82.
- Amid P. Classification of biomaterials and their related complications in abdominal wall surgery. Hernia 1997;1:15-21. [Crossref]
- Coda A, Lamberti R, Martorana S. Classification of prosthetics used in hernia repair based on weight and biomaterial. Hernia 2012;16:9-20. [Crossref] [PubMed]
- Weyhe D, Schmitz I, Belyaev O, et al. Experimental comparison of monofile light and heavy polypropylene meshes: less weight does not mean less biological response. World J Surg 2006;30:1586-91. [Crossref] [PubMed]
- Klinge U, Klosterhalfen B, Ottinger AP, et al. PVDF as a new polymer for the construction of surgical meshes. Biomaterials 2002;23:3487-93. [Crossref] [PubMed]
- Berger D, Bientzle M. Polyvinylidene fluoride: a suitable mesh material for laparoscopic incisional and parastomal hernia repair! Hernia 2009;13:167-72. [Crossref] [PubMed]
- Deeken CR, Faucher KM, Matthews BD. A review of the composition, characteristics, and effectiveness of barrier mesh prostheses utilized for laparoscopic ventral hernia repair. Surg Endosc 2012;26:566-75. [Crossref] [PubMed]
- Deeken CR, Abdo MS, Frisella MM, et al. Physicomechanical evaluation of polypropylene, polyester, and polytetrafluoroethylene meshes for inguinal hernia repair. J Am Coll Surg 2011;212:68-79. [Crossref] [PubMed]
- Saberski ER, Orenstein SB, Novitsky YW. Anisotropic evaluation of synthetic surgical meshes. Hernia 2011;15:47-52. [Crossref] [PubMed]
- Anurov MV, Titkova SM, Oettinger AP. Impact of position of light mesh endoprosthesis with anisotropic structure for the efficiency of anterior abdominal wall reconstruction. Bull Exp Biol Med 2010;149:440-4. [Crossref] [PubMed]
- Cobb WS, Peindl RM, Zerey M, et al. Mesh terminology 101. Hernia 2009;13:1-6. [Crossref] [PubMed]
- Klinge U, Klosterhalfen B. Modified classification of surgical meshes for hernia repair based on the analyses of 1,000 explanted meshes. Hernia 2012;16:251-8. [Crossref] [PubMed]
- Langenbeck MR, Schmidt J, Zirngibi H. Comparison of biomaterials in the early postoperative period: polypropylene meshes in laparoscopic inguinal hernia repair. Surg Endosc 2003;17:1105-9. [Crossref]
- Kossovy N, Freiman CJ, Howarth D. Biomaterials pathology. In: Bendavid R, Abrahamson J, Arregui ME, et al. editors. Abdominal wall hernias, principles and management. New York: Springer-Verlag, 2001;225.
- Schumpelick V, Klinge U, Rosch R, et al. Light weight meshes in incisional hernia repair. J Minim Access Surg 2006;2:117-23. [Crossref] [PubMed]
- Novitsky YW, Harrell AG, Hope WW, et al. Meshes in hernia repair. Surg Technol Int 2007;16:123-7. [PubMed]
- Cobb WS, Kercher KW, Heniford BT. The argument for lightweight polypropylene mesh in hernia repair. Surg Innov 2005;12:63-9. [Crossref] [PubMed]
- Champault G, Barrat C. Inguinal hernia repair with beta glucan-coated mesh: results at two-year follow up. Hernia 2005;9:125-30. [Crossref] [PubMed]
- Losanoff JE, Richman BW, Jones JW. Entero-colocutaneous fistula: a late consequence of polypropylene mesh abdominal wall repair: case report and review of the literature. Hernia 2002;6:144-7. [Crossref] [PubMed]
- Chuback JA, Sigh RS, Sill D, et al. Small bowel obstruction resulting from mesh plug migration after open inguinal hernia repair. Surgery 2000;127:475-6. [Crossref] [PubMed]
- Bellón JM, Rodríguez M, García-Honduvilla N, et al. Peritoneal effects of prosthetic meshes use to repair abdominal wall defects: monitoring adhesions by sequential laparoscopy. J Laparoendosc Adv Surg Tech A 2007;17:160-6. [Crossref] [PubMed]
- Langer C, Schwartz P, Krause P, et al. In-vitro study of the cellular response of human fibroblasts cultured on alloplastic hernia meshes. Influence of mesh material and structure. Chirurg 2005;76:876-85. [Crossref] [PubMed]
- Gumargalieva KZ, Mosieev YV, Daurova TT, et al. Effects of infections on the degradation of polyethylene terephthalate implants. Biomaterials 1982;3:177-80. [Crossref] [PubMed]
- Klinge U, Klosterhalfen B, Muller M, et al. Influence of polyglactin-coating on functional and morphologic parameters of polypropylene-mesh modifications for abdominal wall repair. Biomaterials 1999;20:613. [Crossref] [PubMed]
- Scheidbach H, Tamme C, Tanapfel A, et al. In vivo studies comparing the biocompatibility of various polypropylene meshes and their handling properties during endoscopic total extraperitoneal (TEP) patchplasty. An experimental study in pigs. Surg Endosc 2004;18:211-20. [Crossref] [PubMed]
- Brown CN, Finch JG. Which mesh for hernia repair? Ann R Coll Surg Engl 2010;92:272-8. [Crossref] [PubMed]
- O'Dwyer PJ, Kingsnorth AN, Molloy RG, et al. Randomized clinical trial assessing impact of a lightweight or heavyweight mesh on chronic pain after inguinal hernia repair. Br J Surg 2005;92:166-70. [Crossref] [PubMed]
- Junge K, Klinge U, Rosch R, et al. Functional and morphologic properties of a modified mesh for inguinal hernia repair offer advantages over nonabsorbable meshes. Am J Surg 2002;26:1472-80.
- Bellón JM, Rodríguez M, García-Honduvilla N, et al. Partially absorbable meshes for hernia repair offer advantages over nonabsorbable meshes. Am J Surg 2007;194:68-74. [Crossref] [PubMed]
- Klinge U. Mesh for hernia repair. British Journal of Surgery 2008;95:539-40. [Crossref] [PubMed]
- Zhukovsky V, Rovinskaya L, Vinokurova T, et al. The Development and Manufacture of Polymeric Endoprosthetic Meshes for the Surgery of Soft Tissues. Autex Res J 2002;2:204-9.
- Tongaonkar RR, Reddy BV, Mehta VK, et al. Preliminary multicentric trial of cheap indigenous mosquito-net cloth for tension-free hernia repair. Indian J Surg 2003;65:89-95.
- Stephenson BM, Kingsnorth AN. Inguinal hernioplasty using mosquito net mesh in low income countries: an alternative and cost effective prosthesis. BMJ 2011;343:d7448. [Crossref] [PubMed]
- Clarke MG, Oppong C, Simmermacher R, et al. The use of sterilised polyester mosquito net mesh for inguinal hernia repair in Ghana. Hernia 2009;13:155-9. [Crossref] [PubMed]
- Sanders DL, Kingsnorth AN, Stephenson BM. Mosquito net mesh for abdominal wall hernioplasty: a comparison of material characteristics with commercial prosthetics. World J Surg 2013;37:737-45. [Crossref] [PubMed]
- Sørensen CG, Rosenberg J. The use of sterilized mosquito nets for hernioplasty: a systematic review. Hernia 2012;16:621-5. [Crossref] [PubMed]
- Löfgren J, Nordin P, Ibingira C, et al. A randomized trial of low-cost mesh in groin hernia repair. N Engl J Med 2016;374:146-53. [Crossref] [PubMed]
- ClinicalTrials.gov [Internet]. Bethesda (MD): National Library of Medicine (US). 2000 Feb 29 -. Identifier NCT03324854, Use of Mosquito Net Mesh in Comparison With Polypropylene Mesh for Ventral Hernia Repair; 2006 Feb 3 [cited 2018 Sept 03]; [about 4 screens]. Available online: https://clinicaltrials.gov/ct2/show/NCT03324854
- Salgaonkar H, Lomanto D. Mesh technology. Ann Laparosc Endosc Surg 2017;2:116. [Crossref]
Cite this article as: Salgaonkar H, Lomanto D. Mesh technology—an update. Ann Laparosc Endosc Surg 2018;3:82.