Use of fluoroscopy in endoscopy: indications, uses, and safety considerations
Indications/uses
Historically, fluoroscopy was a tool of the radiologist. Interventional cardiologists and vascular surgeons have revolutionized their respective fields by adopting and adapting its use to their respective practice. This expansion of fluoroscopic utilization has also flourished within the field of endoscopy and continues to evolve.
Perhaps the most common use of fluoroscopy in the endoscopy suite is endoscopic retrograde cholangiopancreatography (ERCP). This procedure is commonly used in the management of common bile duct stones, malignant obstructive jaundice, and post-operative bile leaks. A diagnostic ERCP uses a side-viewing duodenoscope to cannulate the common bile duct with a guidewire under fluoroscopic guidance, followed by passage of a catheter through which contrast is injected to complete the cholangiogram (Figure 1). From there, numerous therapeutic interventions can be performed, including biliary and pancreatic stent placement, biopsy brushings, and balloon sweeps on the bile duct to remove stones and debris. Fluoroscopy has afforded this great asset in management of these biliary-pancreatic conditions.
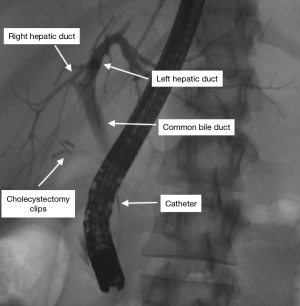
A growing indication for fluoroscopy in endoscopy is the placement of enteral stents. These include esophageal, gastric, duodenal, and colonic stents used in the setting of advanced malignancies for palliative restoration of luminal patency. Off-label use is expanding rapidly to address benign conditions including strictures and leaks. Although, ongoing investigation is needed to evaluate relative efficacy compared to standard surgical interventions. Fluoroscopy guides placement of these stents, monitoring for intra-operative and post-operative complications (i.e., stent migration, perforation).
The Food and Drug Administration (FDA) originally approved esophageal stents for use in stricture and/or fistula related to malignant disease, and remains the preferred palliative intervention for dysphagia and fistulae in patients with esophageal cancer (1). Fully covered Polyflex self-expandable stents (Boston Scientific, Natick, MA) are also FDA-approved for benign refractory esophageal strictures (2). Off-label, but common usages for self-expandable stents include benign esophageal disorders such as tracheoesophageal fistulas, benign esophageal strictures, esophageal perforations, and leaks. Although esophageal stents may be safely placed with only endoscopic guidance, fluoroscopy is a reliable method for placing stents across strictures that are not amenable to dilation or scope passage (3,4). Esophageal stents are placed using thick, semi-rigid delivery systems and utilize a guidewire for safe placement. An endoscope can be used alongside the stent for direct visualization, to accurately assess the length of the obstruction, and to mark the proximal and distal extent. Endoscopic placement of clips, radiocontrast injection in the submucosa, and extracorporeal marking are all methods that assist in accurate placement of the stent while avoiding the upper and lower esophageal sphincters (5). Self-expanding plastic stents include barium markings in both ends and the middle for ease of fluoroscopic placement, and are being used for benign conditions (6).
Gastric outlet obstruction due to unresectable malignancy (i.e., gastric cancer, pancreatic adenocarcinoma, etc.) is the most common indication for placement of a gastroduodenal stent. Although randomized control trial data presents mixed results, current data from non-randomized trials comparing open surgical gastrojejunostomy to endoscopic placement self-expanding metallic stents favors stenting due to shorter time to tolerating an oral intake, similar rate of complications, lower mortality and a shorter hospital stay (7). Fluoroscopy is crucial for accurate characterization of the stricture and stent placement as the obstruction is often too advanced to allow passage of an endoscope. Furthermore, fluoroscopy can identify the presence of multiple duodenal strictures, which can be common in peritoneal carcinomatosis and a relative contraindication to stenting. The stent is deployed using guidewire and through-the-scope technology when possible (5).
Malignant large bowel obstruction is the most common indication for placement of a colonic stent, and occasionally benign conditions such as diverticular stricture warrant stenting. Fluoroscopy easily demonstrates the dilated colon proximal to the obstruction and monitors for perforation, which is high risk because the colon is often very distended and friable (2). Stent placement may be solely fluoroscopic, but is typically done using a through-the-scope technique with technical and clinical success rates over 85%. Endoscopic stenting under fluoroscopic guidance for acute colonic obstruction may serve as a bridge to a single-stage partial colectomy, potentially sparing patients a two-stage procedure and stoma (8). Without the use of fluoroscopic guidance, particularly when a lesion cannot be traversed with the scope and requires distal injection of contrast via a catheter to confirm luminal placement of the guidewire, this expanding array of clinical applications of stents would not be possible.
Another endoscopic option for management of benign strictures is balloon dilation, which is often accompanied by fluoroscopic guidance. Similar to the principles described above for stent placement, if a stricture is unable to be traversed by the scope (Figure 2A), contrast can be injected distally via a catheter over a guidewire to confirm intraluminal location of the guidewire (Figure 2B). Balloon dilation can then be performed over the guidewire, with contrast inside the balloon to further confirm adequate location of the balloon (Figure 2C). As the balloon is inflated, in addition to monitoring the pressurization of the balloon, the endoscopist can fluoroscopically watch the waste eliminate from the balloon. This is another tool for assuring adequate dilation made possible by fluoroscopy.
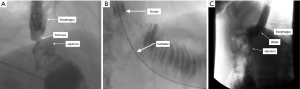
In addition to ERCP, stents and balloon dilation, fluoroscopy is also being used in the management of chronic fistulae of the gastrointestinal tract. This has evolved with advancements in endoscopic techniques including the use of over the scope clips, through-the-scope clips, suturing, stenting, etc. Fluoroscopy is used to identify the fistula tract, and can detect the presence of multiple fistula tracts, as these tracts can be challenging to visualize with endoscopy alone. Once a suspected orifice is identified (Figure 3A), a catheter is used to inject contrast through the orifice to confirm correct location for clip application (Figure 3B). Following deployment of the over the scope clip (Figure 3C), fluoroscopy is again used endoscopically to confirm complete closure. Success rates have been reported as high as 100% for closure of gastrointestinal leaks, and 76% for fistulae (9). As endoscopists increasing gain comfort in the use of fluoroscopy, it is exciting to see how its application will continue to evolve and how these techniques will transform patient care.
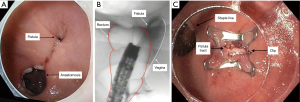
Safety considerations
While the indications for fluoroscopy during endoscopic procedures continue to expand, formal training in radiation exposure and protection is still not widely emphasized during advanced endoscopy training (10). The risks of adverse radiation effects are almost always outweighed by the patient’s benefit of these procedures. However, to improve this risk-to-benefit ratio, especially since only the patient receives the benefit while both the patient and staff assume exposure risk, it is imperative that the operator understands principles behind radiation and how to minimize exposure.
Radiation exposure can result in two major categories of adverse effects: skin injury, and malignancy. These adverse effects are due to the ionizing nature of X-ray radiation, which involves loss of electrons from molecular structures resulting in free radicals. These free radicals can damage DNA. While cells have the ability to repair damaged DNA, if this does not occur, the cell will likely die. Yet, some cells survive the insult and become malignant. High radiation doses typically result in cellular death, as seen with radiation skin damage. Low radiation doses are less likely to kill cells, but may result in malignant changes.
The amount of exposure required to cause skin injury has been described, yet thresholds to cause cancer are less definitive. Acute radiation doses may cause skin erythema at 2 Gy, cataracts at 2 Gy, permanent epilation at 7 Gy, and delayed skin necrosis at 12 Gy (11). The International Commission on Radiologic Protection (ICRP) speculates that the incidence of cancer in all organs among individuals exposed to ionizing radiation increases by 5% per Sievert (12). To minimize this risk of malignancy, it is essential to use radiation levels as low as reasonably achievable (ALARA) for every procedure.
Understanding radiation exposure and potential for carcinogenicity can be challenging for the average physician to interpret and compare, as multiple units for measuring exposure are reported in the literature. Although tedious, it is important to understand these different metrics. Gray is a System International (SI) unit, measured in joule per kilogram, which describes an absorbed dose of radiation. Gray can easily be converted to rads (1 Gy =100 rads). And, a rad is equivalent to a roentgen, an older unit of measure (13). The use of rads and roentgen is often discouraged in academia as they are not part of the SI system. Sieverts is an SI unit, also measured in joule per kilogram, that adjusts the dose absorbed based on a conversion factor—called the radiation weighing factor—that varies according to the specific type of radiation exposure (i.e., photons, electrons, neutrons, alpha particles, etc.). Sieverts and rems (1 Sv =100 rems) measure biologic dose exposure and can describe equivalent dose, effective dose, and operational dose quantities. This is why Sieverts and rems are the preferred units for describing occupational hazard (12).
The average radiation dose to healthcare workers in the United States is 0.3 mSv annually (14). For medical professionals, even small reductions in radiation exposure from improved technique can alter risk of malignancy when extrapolated over the length of a career. Reduction in radiation exposure to self and staff can be accomplished through a variety of practices. First, standing as far away from the X-ray source as possible reduces exposure exponentially. When X-ray is used, the beams have (I) complete penetration, in which the X-ray passes through the tissue into the image-recording device, (II) total absorption, in which the tissue absorbs the X-ray energy, and (III) partial absorption with scatter. Scattered radiation has low energy but widespread trajectory. It is these scattered beams that are the primary source of radiation to the operator and staff.
As X-rays leave the machine, they travel in straight but divergent paths. This spread of X-ray beams increases as the distance from the source increases. Therefore, the number of X-rays traveling through a given area decreases with increasing distance from the source. This principle is the basis of the inverse square law, which describes the degree of radiation exposure reduction caused by divergence. Doubling the distance from a radiation source quarters the radiation exposure. Conversely, halving the distance increases exposure 4-fold. Outside of a 6-foot radius, the radiation exposure has been shown to be negligible (15). Applying these principles by maximizing the distance between staff and the X-ray source can significantly reduce occupational radiation exposure.
When the X-ray tube is below the table, radiation levels are higher beneath the table with the highest levels of radiation directed at the operator’s waist. When using oblique views, the operator’s head and eyes are at higher risk for radiation exposure when the tube is tilted towards the operator. Therefore, in addition to standing as far back as possible, if feasible, the operator should stand on the detector side of the table when acquiring oblique images.
In addition to applying the Inverse Square Law, the physician has control of additional measures to reduce radiation exposure including appropriate use of collimation, a grid, pulsed fluoroscopy, and efficient use of frames. Collimators are lead shutters that help shape the emerging beams of radiation as they exit the X-ray tube. The physician can adjust the collimators to provide beams of different shapes and sizes. This allows the physician to narrow imaging to areas of high diagnostic value and avoid imaging the surrounding tissues. Appropriate use of collimation can reduce the total radiation dose delivered to the patient, as well as the low-energy scatter delivered to the environment. By reducing overall scatter, use of collimation also improves image quality, since some of the scattered X-rays also enter the detector, which degrades the quality of the image. Furthermore, the physician can narrow the field to exclude high-density areas, which improves the resolution of the tissue of interest.
A grid is another tool used to block scattered X-rays. The catch-22 of the grid is that by blocking scatter to the environment, overall fewer X-rays reach the sensor, which increases exposure to the patient via an automatic brightness control. Where this comes into play is with patients with a large air-gap between the fluoroscopy machine and the patient—for example a small-sized or pediatric patient. The large air-gap allows more scatter to be missed by the receptor, which with the use of a grid exponentially increases the exposure. In these patients, it is best to remove the grid.
Utilization of a pulsed mode of fluoroscopy is another tool the physician possesses to minimize radiation (16). This mode generates electrons to flow in short spurts through the X-ray tube rather than continuously. Most modern fluoroscopic devices have this capability and allow the physician to choose the rate of pulsations per second. Total radiation dose has been shown to decrease by 22%, 38%, and 49% at 15, 10, and 7.5 frames per second respectively (17).
The amount of radiation exposure in fluoroscopy is also dependent on the total time the X-ray is activated by the physician. Therefore, reducing the number and length of frames can greatly affect the overall dose delivered to the patient and scatter delivered to bystanders. The physician must be mindful of this at all times. To assist, many machines have an alarm that warns the physician when 5 minutes of fluoroscopy has been reached. Furthermore, the physician can freeze-frame images to review, typically displayed in a side-by-side fashion, while fluoroscopy is not activated. These measures, along with simply releasing the activating foot pedal as soon as the desired images are achieved, are ways in which the physician can reduce radiation exposure to both the patient and staff.
An additional physician-driven means of controlling radiation exposure includes the use of real-time dosimeters. These have been shown to be an effective tool for refining technique and reducing overall radiation via immediate feedback to the operator (18,19). This is particularly useful in training facilities, where radiation exposure is significantly higher (20). As societal guidelines are starting to incorporate quality metrics for the amount of radiation used per procedure—often referred to as the diagnostic reference level—this will serve as another feedback tool by which physicians can measure and improve individual technique (21).
Lastly, a review of radiation safety would not be complete without discussing the importance of personal shielding equipment. Lead aprons block more than 90% of scattered radiation to the body (22). While there have been limited studies to date evaluating the relative efficacy of different lead apron types, wrap-around aprons and vest/skirt combinations theoretically decrease loading stress on the cervical and thoracic spine. Additionally, a front covering apron alone is not adequate if the surgeon will be frequently facing away from the patient (23). In 2009, the World Gastroenterology Organization recommended that wrap-around apron with a suitable lead equivalence of 0.25–0.35 mm and thyroid shield should be worn by everyone in the room, aside from the patient, when using fluoroscopy (16). Compared to age-matched controls, healthcare workers routinely exposed to fluoroscopy are significantly more likely to develop cataracts (24). Clear lead glasses can reduce the ocular radiation exposure by 85–90% (25), and their use is recommended if a protective shield is not already in place (16). Fluoroscopists also experience high levels of radiation exposure to the hands. Options for protecting the hands from radiation exposure include lead gloves and gloves impregnated with contrast agents such as barium sulfate (26). However, use of protective gloves is controversial, as studies have demonstrated that wearing lead gloves increases the time surgeons leave their hands in the field of view (23). In fact, World Gastroenterology Organization Global Guidelines recommend against the use of these gloves, as it is best practice to keep the hands out of the radiation field (16).
In summary, fluoroscopy is a great adjunct to endoscopy and its indications continue to evolve. As this field expands, it is essential that we educate ourselves on the safe use of radiation and minimize exposure.
Acknowledgments
Funding: None.
Footnote
Provenance and Peer Review: This article was commissioned by the editorial office, Annals of Laparoscopic and Endoscopic Surgery for the series “Surgical Endoscopy: Essential Skills in Gastrointestinal Surgery”. The article has undergone external peer review.
Conflicts of Interest: All authors have completed the ICMJE uniform disclosure form (available at http://dx.doi.org/10.21037/ales.2019.06.05). The series “Surgical Endoscopy: Essential Skills in Gastrointestinal Surgery” was commissioned by the editorial office without any funding or sponsorship. JM served as the unpaid Guest Editor of the series. JM has provided consultant services for Olympus and Boston Scientific. The authors have no other conflicts of interest to declare.
Ethical Statement: The authors are accountable for all aspects of the work in ensuring that questions related to the accuracy or integrity of any part of the work are appropriately investigated and resolved.
Open Access Statement: This is an Open Access article distributed in accordance with the Creative Commons Attribution-NonCommercial-NoDerivs 4.0 International License (CC BY-NC-ND 4.0), which permits the non-commercial replication and distribution of the article with the strict proviso that no changes or edits are made and the original work is properly cited (including links to both the formal publication through the relevant DOI and the license). See: https://creativecommons.org/licenses/by-nc-nd/4.0/.
References
- Evans JA, Early DS, Chandraskhara V, et al. The role of endoscopy in the assessment and treatment of esophageal cancer. Gastrointest Endosc 2013;77:328-34. [Crossref] [PubMed]
- Kochar R, Shah N. Enteral stents: from esophagus to colon. Gastrointest Endosc 2013;78:913-8. [Crossref] [PubMed]
- Austin AS, Khan Z, Cole AT, et al. Placement of esophageal self-expanding metallic stents without fluoroscopy. Gastrointest Endosc 2001;54:357-9. [Crossref] [PubMed]
- White RE, Mungatana C, Topazian M. Esophageal stent placement without fluoroscopy. Gastrointest Endosc 2001;53:348-51. [Crossref] [PubMed]
- Adler DG. The role of fluoroscopy in the endoscopic management of luminal gastrointestinal disorders. Tech Gastrointest Endosc 2007;9:189-94. [Crossref]
- Sharma P, Kozarek RPractice Parameters Committee of the American College of Gastroenterology. Role of esophageal stents in benign and malignant diseases. Am J Gastroenterol 2010;105:258-73. [Crossref] [PubMed]
- Nagaraja V, Eslick GD, Cox MR. Endoscopic stenting versus operative gastrojejunostomy for malignant gastric outlet obstruction—a systematic review and meta-analysis of randomized and non-randomized trials. J Gastrointest Oncol 2014;5:92-8. [PubMed]
- Baron TH, Song LM, Repici A. Role of self-expandable stents for patients with colon cancer (with videos). Gastrointest Endosc 2012;75:653-62. [Crossref] [PubMed]
- Winder JS, Kulaylat AN, Schubart JR, et al. Management of non-acute gastrointestinal defects using the over-the-scope clips (OTSCs): a retrospective single-institution experience. Surg Endosc 2016;30:2251-8. [Crossref] [PubMed]
- Sethi S, Barakat MT, Friedland S, et al. Radiation training, radiation protection and fluoroscopy utilization practices among US therapeutic endoscopists. Dig Dis Sci 2019; [Epub ahead of print]. [Crossref] [PubMed]
- Valentin J. Avoidance of radiation injuries from medical interventional procedures. Ann ICRP 2000;30:7-67. [Crossref] [PubMed]
- ICRP 2007. The 2007 recommendations of the International Commission on Radiological Protection. ICRP publication 103. Ann ICRP 2007;37:1-332.
- Singer G. Occupational radiation exposure to the surgeon. JAAOS 2005;13:69-76. [Crossref] [PubMed]
- UNSCEAR. The United Nations Scientific Committee on the Effects of Atomic Radiation. Health Phys 2000;79:314. [PubMed]
- Kendrick DE, Miller CP, Moorehead PA. Comparative occupational radiation exposure between fixed and mobile imaging systems. J Vasc Surg 2016;63:190-7. [Crossref] [PubMed]
- World Gastroenterology Organisation 2009. Radiation protection in the endoscopy suite: minimizing radiation exposure for patients and staff in endoscopy: a joint ASGE/AEA/WGO guideline. Accessed online: http://www.worldgastroenterology.org/guidelines/global-guidelines/radiation- protection-in-the-endoscopy-suite/radiation-protection-in-the-endoscopy-suite-english
- Aufrichtig R, Xue P, Thomas CW, et al. Perceptual comparison of pulsed and continuous fluoroscopy. Med Phys 1994;21:245-56. [Crossref] [PubMed]
- Baumgartner R, Libuit K, Ren D, et al. Reduction of radiation exposure from c-arm fluoroscopy during orthopaedic trauma operations with introduction of real-time dosimetry. J Orthop Trauma 2016;30:e53-8. [Crossref] [PubMed]
- Müller MC, Welle K, Strauss A, et al. Real-time dosimetry reduces radiation exposure of orthopaedic surgeons. Orthop Traumatol Surg Res 2014;100:947-51. [Crossref] [PubMed]
- Bernardi G, Padovani R, Trianni A, et al. The effect of fellows’ training in invasive cardiology on radiological exposure of patients. Radiat Prot Dosimetry 2008;128:72-6. [Crossref] [PubMed]
- Hayashi S, Takenaka M, Hosono M, et al. Radiation exposure during image-guided endoscopic procedures: The next quality indicator for endoscopic retrograde cholangiopancreatography. World J Clin Cases 2018;6:1087-93. [Crossref] [PubMed]
- Pedrosa MC, Farraye FA, Shergill AK, et al. Minimizing occupational hazards in endoscopy: personal protective equipment, radiation safety, and ergonomics. Gastrointest endosc 2010;72:227-35. [Crossref] [PubMed]
- Meisinger QC, Stahl CM, Andre MP, et al. Radiation protection for the fluoroscopy operator and staff. AJR Am J Roentgenol 2016;207:745-54. [Crossref] [PubMed]
- Vano E, Kleiman NJ, Duran A, et al. Radiation-associated lens opacities in catheterization personnel: results of a survey and direct assessments. J Vasc Interv Radiol 2013;24:197-204. [Crossref] [PubMed]
- Burns S, Thornton R, Dauer LT, et al. Leaded eyeglasses substantially reduce radiation exposure of the surgeon’s eyes during acquisition of typical fluoroscopic views of the hip and pelvis. J Bone Joint Surg Am 2013;95:1307-11. [Crossref] [PubMed]
- Kayan M, Yaşar S, Saygın M, et al. Investigation of x-ray permeability of surgical gloves coated with different contrast agents. Anatol J Cardiol 2016;16:424-7. [PubMed]
Cite this article as: Sippey M, Maskal S, Anderson M, Marks J. Use of fluoroscopy in endoscopy: indications, uses, and safety considerations. Ann Laparosc Endosc Surg 2019;4:59.